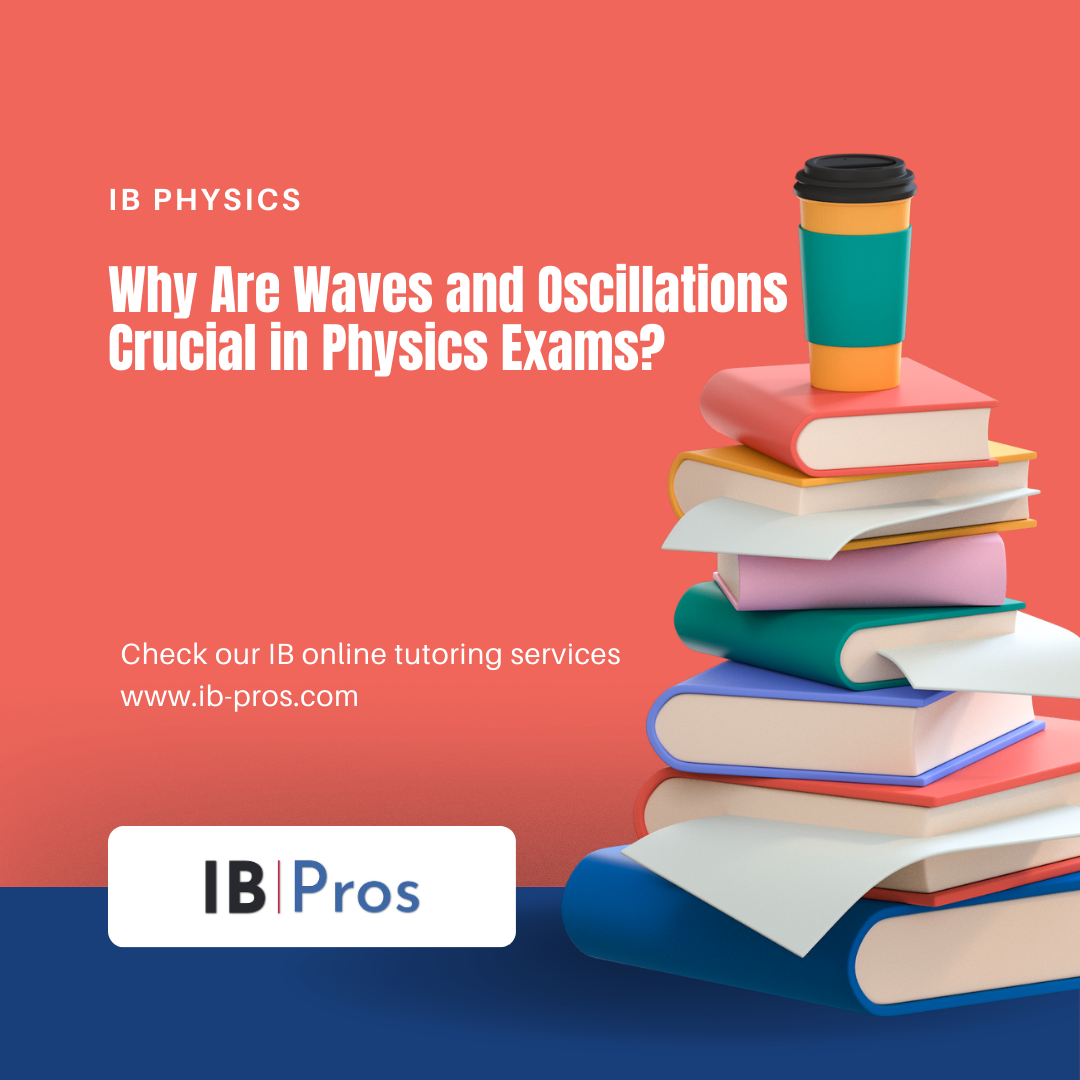
In the realm of physics education, the inclusion of waves and oscillations in standardized examinations is not merely a pedagogical preference but a reflection of their foundational role in understanding the physical world. These phenomena are pervasive, underpinning a diverse array of concepts from the simple pendulum to the complexities of quantum mechanics. By probing students’ comprehension of waves and oscillations, educators can assess not only their grasp of these fundamental principles but also their mathematical dexterity and ability to apply abstract concepts to concrete problems. Moreover, these topics serve as a bridge to more advanced theories, necessitating a robust conceptual framework that students must demonstrate. As we consider the integral functions that waves and oscillations play in the tapestry of physical laws, one must ponder the implications of their significance in shaping a physicist’s educational journey and the broader impact on scientific literacy. What remains to be uncovered is how this focus influences the development of problem-solving skills that are essential for a flourishing career in the sciences.
Key Takeaways
- Waves and oscillations are fundamental concepts in physics exams.
- Mastering waves and oscillations requires mathematical proficiency and problem-solving skills.
- Understanding energy transfer principles is pivotal for analyzing wave behavior.
- Waves and oscillations have connections to advanced theories such as quantum mechanics and general relativity.
Understanding Fundamental Concepts
To truly grasp the complexities of waves and oscillations, one must first establish a firm understanding of their fundamental concepts, including the definitions and differences between various types of waves, the parameters that describe oscillatory motion, and the underlying principles that govern their behavior. Waves, in their most basic form, are disturbances that transfer energy from one point to another without the permanent displacement of the medium through which they travel. They can be classified as mechanical or electromagnetic, longitudinal or transverse, each with distinct characteristics and modes of propagation.
Oscillations refer to the repeated back-and-forth motion of particles about a position of equilibrium, typified by parameters such as amplitude, frequency, period, and phase. These parameters not only quantify the motion but also provide insights into the energy and dynamics of the system under consideration.
The mathematical treatment of waves and oscillations involves a synthesis of these concepts, often employing differential equations to model their behavior. With waves, the wave equation serves as a cornerstone, linking the spatial and temporal progression of the wave with its velocity and wavelength. Oscillatory systems frequently hinge on the principles of simple harmonic motion, resonating with the elegance of sines and cosines to depict the periodic nature of their movement.
In essence, a robust comprehension of these principles is indispensable for tackling physics examinations, which frequently test one’s ability to analyze, predict, and apply wave and oscillatory phenomena to a variety of physical situations.
Demonstrating Mathematical Proficiency
Building upon the foundational knowledge of waves and oscillations, students must now demonstrate their mathematical proficiency through rigorous problem-solving and equation manipulation. Physics exams necessitate a command over various mathematical tools to interpret and predict the behavior of physical systems. This proficiency is not merely a byproduct of rote learning; it stems from a deep comprehension of the principles governing wave phenomena and their mathematical representations.
Students are required to engage with differential equations, perform Fourier analyses, and apply boundary conditions to solve complex wave problems. They must translate physical situations into mathematical language, demonstrating an ability to move seamlessly between abstract mathematical concepts and tangible physical phenomena.
The analytical aspect of this endeavor requires breaking down complex waveforms into simpler harmonic components, a skill that underpins the field of signal processing and acoustics among others. An authoritative grasp over these mathematical techniques ensures that students are not just passive recipients of information, but active analyzers and synthesists of data.
Pedagogically, the focus on mathematical proficiency in the context of waves and oscillations ensures that students are equipped with the necessary skills to tackle advanced topics in physics and engineering. This rigor in mathematical training is indispensable for academic progression and professional success within scientific disciplines.
Exploring Energy Transfer
Delving into the realm of energy transfer, we scrutinize the mechanisms by which waves propagate energy through various media, a pivotal concept in understanding the dynamic processes that underlie oscillatory systems. Waves serve as the conduits of energy, rather than the transporters of matter, enabling phenomena such as sound transmission and electromagnetic radiation. In physics examinations, the analysis of energy transfer through waves demands a rigorous understanding of wave characteristics and the principles governing their behavior.
Mechanical waves, such as sound and water waves, necessitate a physical medium to convey energy, transferring it via particle interactions. Contrastingly, electromagnetic waves, including light and radio waves, can transmit energy across the vacuum of space, facilitated by oscillating electric and magnetic fields. These distinctions are crucial for students to navigate complex problems and develop a robust comprehension of wave mechanics.
The pedagogical emphasis on energy transfer is thus twofold: first, to impart a foundational knowledge of the types of waves and their respective modes of energy propagation; second, to equip students with the analytical skills to apply conservation of energy principles within wave phenomena. Mastery of these concepts is indispensable, as it not only underpins various scientific disciplines but also fosters critical thinking and problem-solving skills essential for academic success.
Connecting to Advanced Theories
As students progress beyond foundational wave mechanics, they encounter advanced theories that elucidate the intricate connections between waves, particles, and the fundamental forces of nature. The study of waves and oscillations is not confined to classical mechanics; it extends into quantum mechanics, where the wave-particle duality becomes a cornerstone concept. The Schrödinger equation, for instance, characterizes the behavior of particles as wavefunctions, with solutions that describe probability amplitudes for particle positions and momenta. Mastery of wave behavior is thus imperative for understanding atomic and subatomic scales.
Furthermore, the framework of quantum field theory (QFT) is predicated upon the oscillations of quantum fields. Particles are excitations of these fields, much like waves propagating through a medium. This view is critical for comprehending the interactions described by the Standard Model of particle physics, which explains how force-carrying particles – the bosons – mediate the fundamental forces among fermions, the matter constituents. Waves and oscillations are also pivotal in general relativity, where gravitational waves—ripples in spacetime itself—are a direct consequence of Einstein’s field equations. Given their ubiquity across multiple scales and systems, waves and oscillations form an essential component of the theoretical apparatus of physics, and their study is crucial for students aiming to navigate the depths of the discipline.
Cultivating Problem-Solving Skills
Having established the theoretical importance of waves and oscillations across various domains of physics, it is imperative to focus on developing the problem-solving skills necessary to apply these concepts effectively. Mastery in problem-solving not only entails understanding the underlying principles but also involves the ability to dissect complex scenarios into solvable components. This proficiency is honed through structured practice and exposure to a variety of problems.
Key SkillDescriptionExample ApplicationConceptual UnderstandingGrasping the fundamental principles of waves and oscillations.Analyzing wave interference patterns.Mathematical FormulationTranslating physical situations into mathematical equations.Calculating the frequency of oscillating systems.Analytical ReasoningBreaking down problems into manageable parts.Determining the movement of a particle in a wave.Logical SynthesisCombining individual insights to form a coherent solution.Solving for wave speed in a medium.Practical ApplicationApplying theoretical knowledge to real-world situations.Designing experiments to test wave properties.
Students must be encouraged to engage with problems analytically, seeking not only the correct solution but also a deep understanding of the processes involved. This involves recognizing patterns, applying appropriate formulas, and critically evaluating results. Moreover, a pedagogical approach that emphasizes the interconnectivity of physics concepts will facilitate a more comprehensive grasp of the subject matter, thereby advancing students’ ability to tackle complex wave and oscillation problems with confidence and precision.
Frequently Asked Questions
How Do Waves and Oscillations Relate to Real-World Engineering Problems and Solutions?
Waves and oscillations are fundamental to addressing real-world engineering challenges. They manifest in various phenomena, from the resonant frequencies of bridges to the electromagnetic waves used in telecommunications. Engineers must understand and manipulate these concepts to design stable structures, improve signal transmission, and develop innovative technologies like noise-cancelling headphones. Mastery of wave dynamics is essential for creating solutions that are efficient, safe, and in harmony with the natural environment.
Can You Provide Historical Examples of How the Study of Waves and Oscillations Has Led to Technological Breakthroughs?
Historical examination of waves and oscillations reveals profound technological advancements. Hertz’s discovery of electromagnetic waves preluded wireless communication; understanding seismic oscillations has enhanced earthquake-resistant structures. Additionally, the study of sound waves facilitated the development of acoustic devices. These instances underscore the critical role of wave and oscillation research in driving innovation, reflecting its foundational importance in the evolution of contemporary technology and its integration into modern society’s fabric.
Are There Any Common Misconceptions Students Have About Waves and Oscillations That Could Hinder Their Understanding?
Students often misconceive waves and oscillations, viewing them as abstract concepts rather than fundamental phenomena. A common misunderstanding is equating wave velocity with particle motion, overlooking that particles in a medium typically oscillate in place. Additionally, the distinction between transverse and longitudinal waves can be confusing, with students struggling to visualize how the latter propagate. Such misconceptions can impair the comprehension of wave mechanics, a cornerstone in diverse fields like acoustics, optics, and quantum physics.
How Can Interdisciplinary Studies Enhance a Student’s Grasp of Waves and Oscillations in Physics?
Interdisciplinary studies significantly bolster comprehension of waves and oscillations by providing diverse perspectives and applications that reinforce core physics principles. Exposure to disciplines such as mathematics, engineering, and computer science allows students to see the practical implications and theoretical underpinnings, leading to a more robust understanding. This multifaceted approach fosters critical thinking and problem-solving skills essential for mastering complex concepts within the realm of physics.
What Are the Most Effective Revision Techniques for Retaining Information About Waves and Oscillations for Long-Term Memory?
Effective revision techniques for long-term retention of information on waves and oscillations include spaced repetition, active recall, and the Feynman Technique. Spaced repetition leverages increasing intervals of time between review sessions to enhance memory, while active recall involves testing oneself on the material. The Feynman Technique requires explaining concepts in simple terms, which aids in deepening understanding and uncovering any gaps in knowledge. Consistent application of these methods fosters robust memory retention.
Conclusion
In conclusion, waves and oscillations represent fundamental principles that bridge multiple domains within physics, providing a foundation for understanding complex phenomena. Proficiency in these topics not only demonstrates mathematical acumen but also facilitates comprehension of energy transfer mechanisms, which are pivotal in various physical systems. Moreover, they serve as precursors to advanced theoretical constructs, while honing analytical and problem-solving skills, thereby underscoring their significance in physics education and examinations.